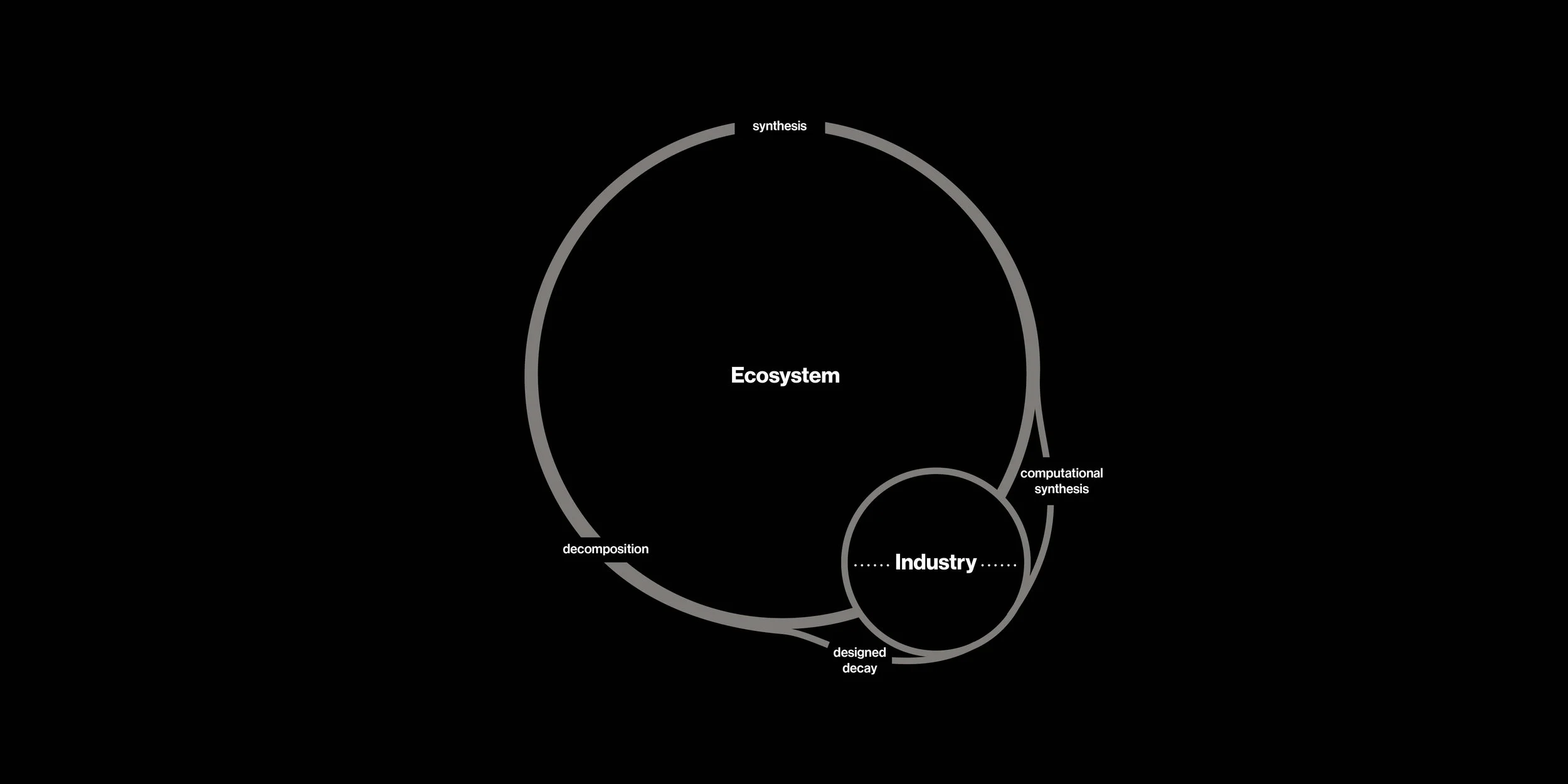
Endless Ecosystems is a design framework that leverages the power of biodiverse ecosystems to produce and recycle raw materials –
in essence enabling systems of production without waste.
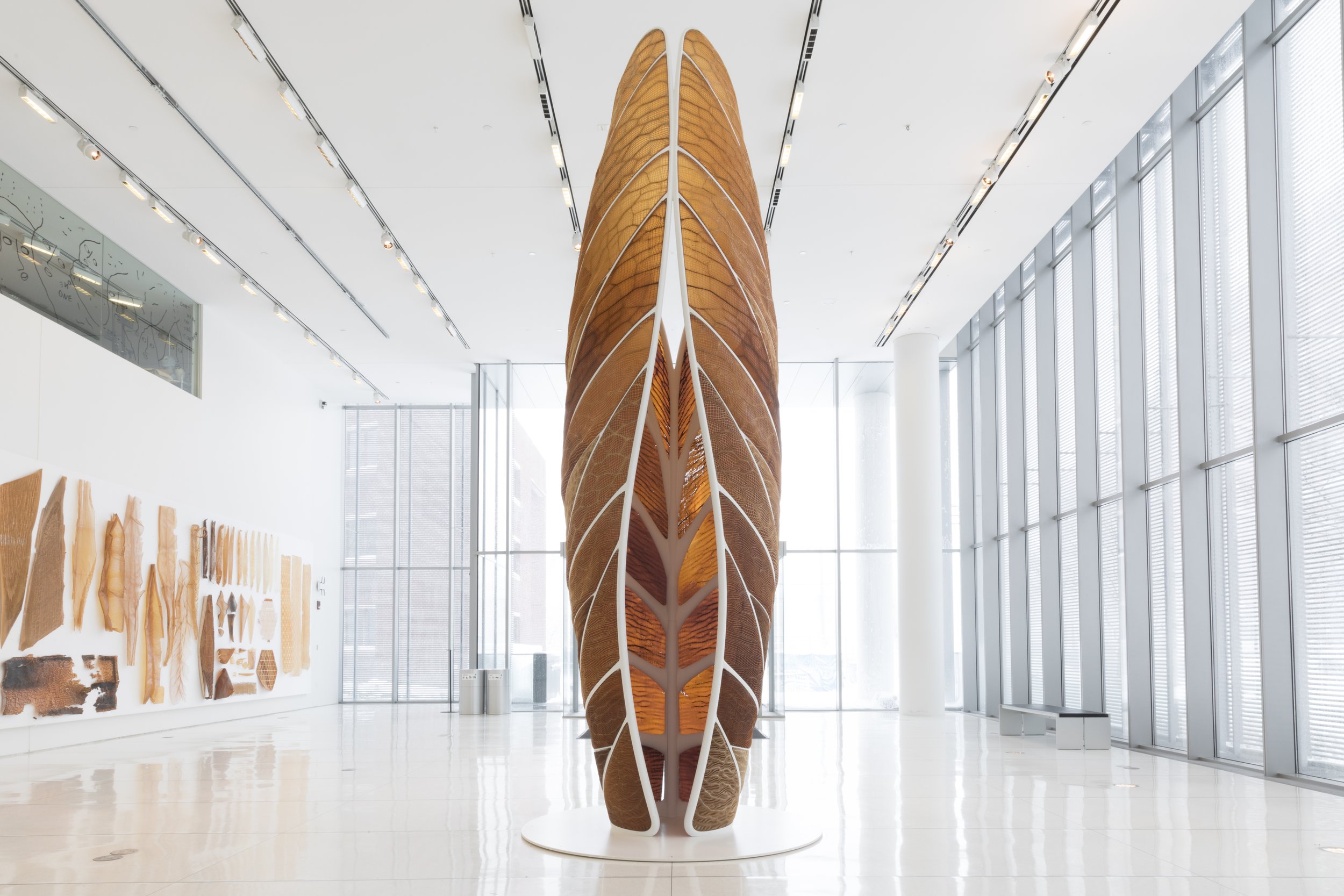
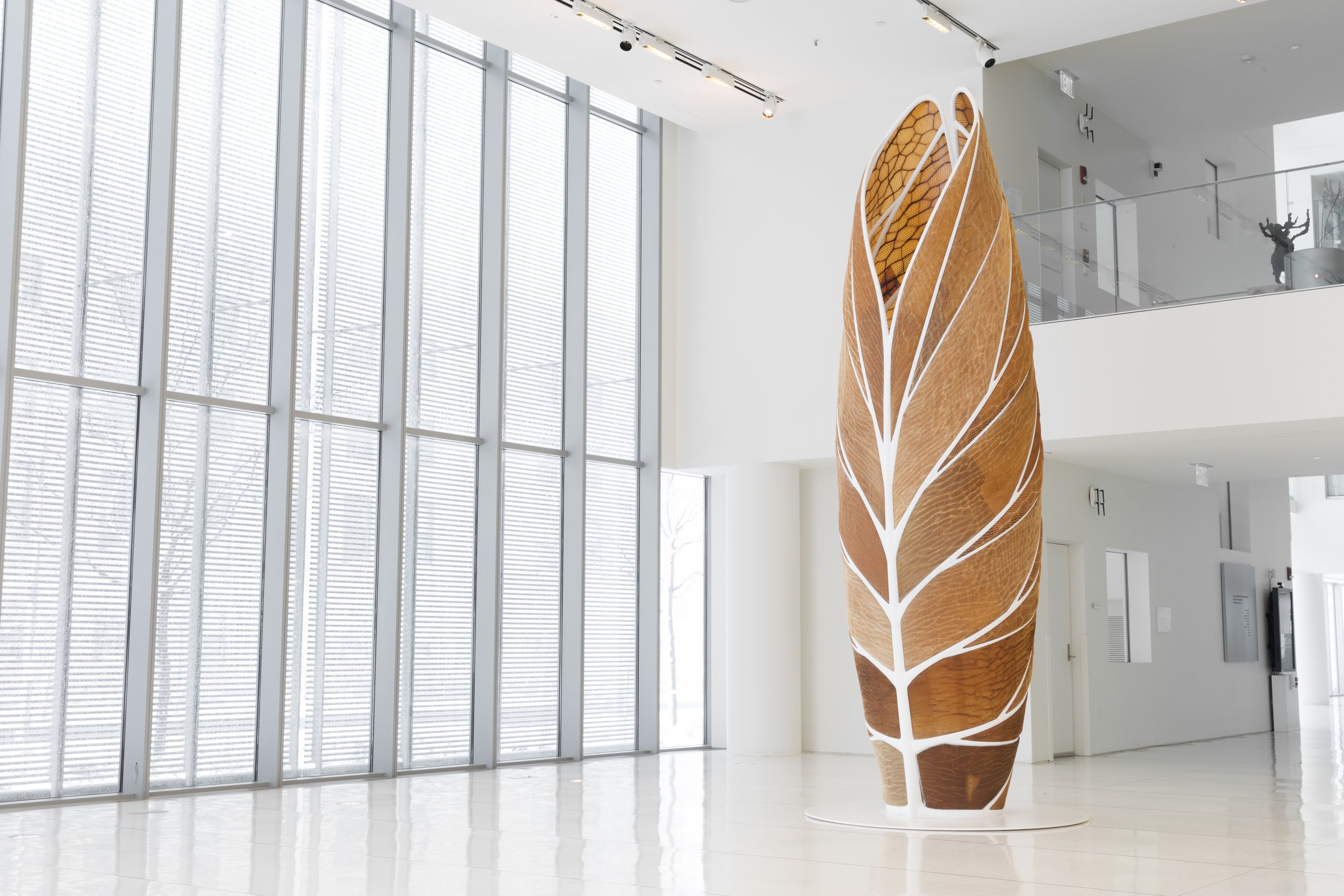
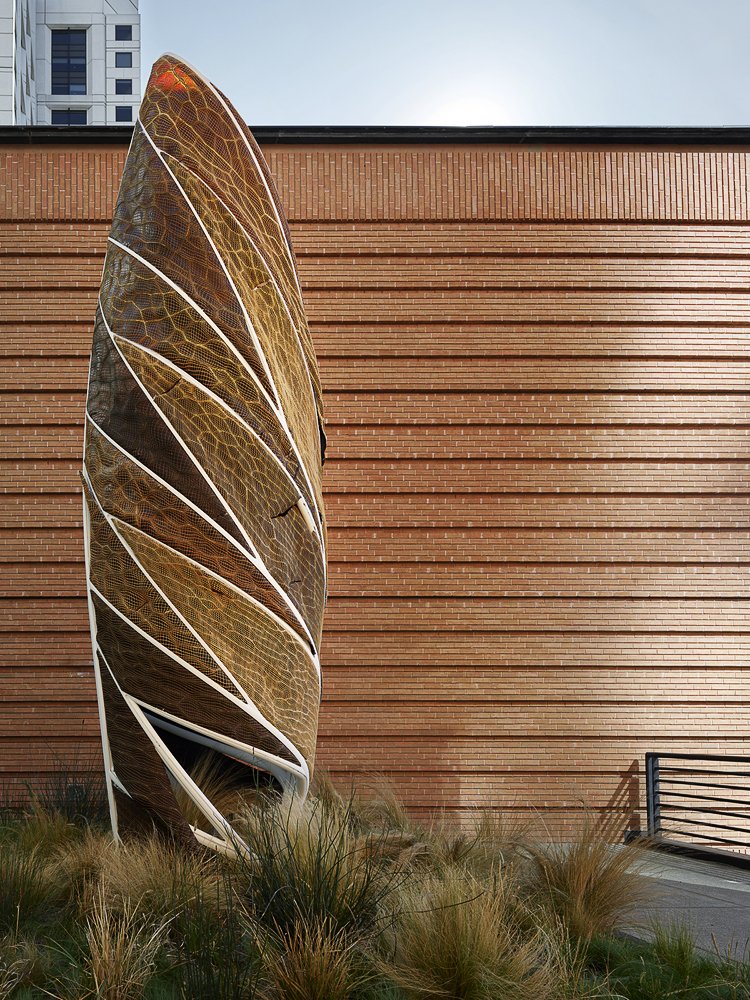
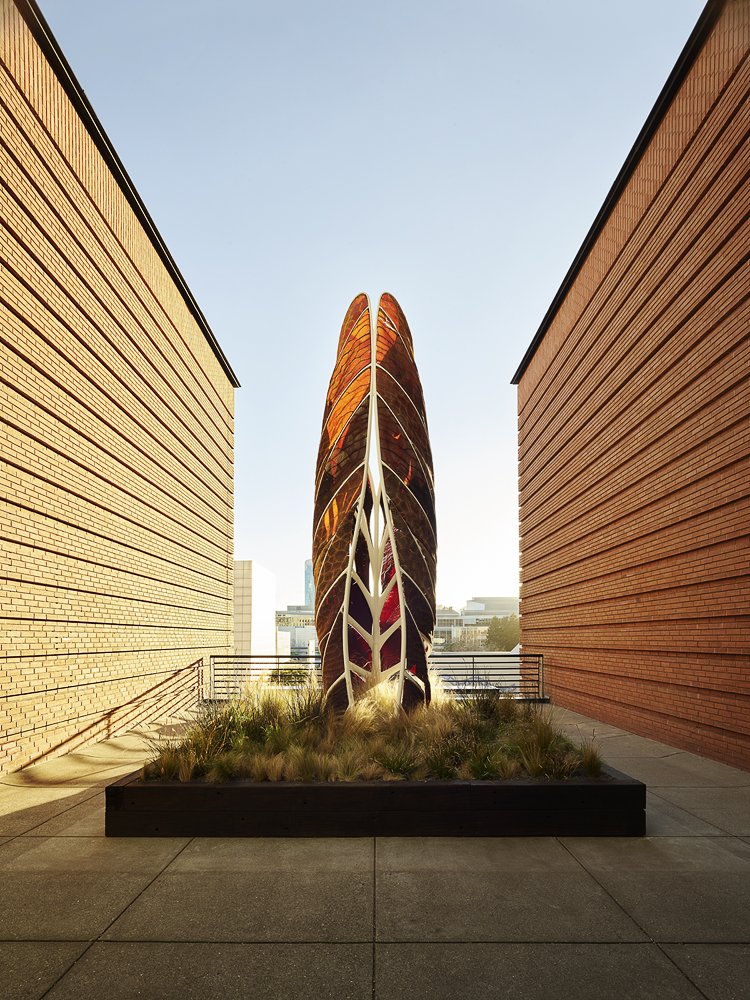
Overview
According to the United Nations Climate Programme, over 300 million tons of plastic are produced every year. Less than one tenth of this material is recycled, with vast quantities being transported to landfills or circulating ocean currents. By contrast, ecosystems produce and consume matter in a cycle where waste is virtually nonexistent. Natural materials are grown from local abundant resources and their death provides fuel for new growth. For humans to mirror nature in this manner would require a shift not only in the materials we use, but in our fundamental notions of design.
My research combines tools from digital design and fabrication, materials science, and energy and environmental analysis to investigate the hypothesis that non-human ecosystems provide enough matter for the production and decomposition of all human construction – in essence creating a world without waste. This journey of matter from synthesis to design, from death to regrowth constitutes an Endless Ecosystem. In this way, the Endless Ecosystem illuminates the fundamental truth that humans, like all other organisms, are inextricably entangled in and dependent on the biosphere.
In practice, two key processes enable the design within the Endless Ecosystems framework. The first is Computational Synthesis in which organic matter is minimally processed to meet the needs of a particular design. The second is Designed Decay by which the energy stored within materials is fed back into non-human ecosystems to reinforce non-human systems of production. Lastly, E(m)ergy Evaluation is applied as a means by which the system’s environmental impact can be quantified and understood.
An application of the Endless Ecosystems framework to the design and analysis of Aguahoja I is outlined below.
Computational Synthesis
From a relatively small palette of molecular components, natural systems construct a vast array of functional materials with no synthetic parallels. Chitin manifests as both thin, transparent dragonfly wings and the rigid exoskeletons of lobsters. The immensely strong wood of a redwood tree and the flexible leaves of the smallest fern are both made from cellulose. Such materials, and the living systems they grow within, frequently outperform human engineering in their diverse functions, resilience, and sustainability.
Computational synthesis is the method by which abundant stocks of organic material are transformed and reshaped in order to meet design constraints. This may involve combining multiple materials to create composites with new capabilities or architecting geometry to achieve specific mechanical properties. In contrast to conventional approaches creating sustainable materials, computational synthesis seeks to begin with materials that exist within the sustainable systems of production and recycling created by ecosystems and meet design constraints without sacrificing these capabilities.
In the design of Aguahoja I, the abundant biopolymers cellulose, chitin, and pectin were used to create composites with tailored mechanical properties. Some achieving the system’s structural requirements required chemical modifications, such as the combination of materials in hydrogel form, as well as geometric modifications, such as the creation of a generative reinforcement pattern of chitosan and cellulose along the structure’s skin. Importantly, these modifications do not impact the ability of the organic composites to be decomposed and digested by other organisms.
Designed Decay
Non-human ecosystems enable sustainable processes not through the minimization of waste by any individual component, but by the consumption of that excess by other components. Within an old forest, deciduous trees may produce hundreds of kilograms of waste in the form of fallen leaves. These leaves are then decomposed by fungi and soil bacteria that, in turn, go on to fix nutrients that enhance the growth of those same trees.
Designed Decay proposes that the death and decomposition of a structure or product are as important an aspect of its design as is its construction and life. By identifying channels through which materials components can either be reused, repaired, or decomposed to fuel new growth, Designed Decay creates circular flows of material.
In practice, this is accomplished by leveraging material stocks that can already be decomposed by non-human ecosystems. This process also requires that the decomposition kinetics of these materials be modeled and well understood so that system components can be decomposed after they have met their use and not before.
Energy Systems Modeling
The Endless Ecosystems framework proposes that designers with the ability to specify where matter comes from, how it is transformed, and where it is disposed of, have the ability to empower non-human ecosystems and create sustainable systems of production. In order to accomplish this, the design of a system is modeled using the ecological systems diagramming approach proposed by Howard Odum which traces renewable and non-renewable inputs to the system and its final outputs.
Within this system, feedback flows can be identified that enable cooperation with ecosystems. Products that can be decomposed and digested by other organisms are accounted for as positive feedback flows that reinforce the system of production, while industrial processes that degrade non-human systems are designated as negative feedback flows.
This system diagram serves as a first step through which renewable resources and positive feedback flows can be maximally leveraged. In the design of Aguahoja I, the use of minimally refined pectin and cellulose in the 3D printed composites both increased the use of renewable sources of energy and provided a means of positive feedback through designed decay.
E(m)ergy Evaluation
Originally developed by the ecologist Howard Odum, Emergy (a contraction of energy and memory) evaluation aims to account for the sum total of energy required to create a product or service. Emergy evaluation serves as a critical tool in the Endless Ecosystems framework by accounting for the work done by non-human systems to produce and decompose raw materials. Currently, other methods such as Life-Cycle assessment do not account for non-human inputs of this nature.
Emergy is quantified in units of solar emjoules (sej), which equate to 1 joule of solar energy. The quantification of all energetic inputs to the designed system serves the dual purpose of requiring a detailed of where all of the matter used by a system originates from and is destined for.
Emergy Hierarchy
Once quantified, this data can be organized into an emergy hierarchy of system components. These hierarchies indicate which materials and processes contribute the most energy to the system. For example, if computation or digital fabrication processes are required in order to use new materials from organic sources, the energy required to process these materials can be easily compared against other inputs to the system.
The emergy hierarchy can be used in order to determine which inputs contribute system emergy disproportionate to their representation by mass. In some systems, small contributions of highly processed material can contribute large amounts of emergy to the system.
The Emergy hierarchy for Aguahoja I indicates that the energy required for computation and material processing is small compared to the contributions of renewable and ecological inputs. The hierarchy also indicates the disproportionate contribution of refined acetic acid and vegetable glycerin, which are present in small amounts within the structure but represent relatively large energetic sums.
Planetary System Mapping
With the origins of matter accounted for by the emergy evaluation, the system’s planetary reach can be mapped and quantified. This mapping provides designers with an understanding of the global ecologies that they are drawing resources from and directing energy towards. Additionally, metrics such as the system’s linear territory and total mass can provide insights into potential impacts, both positive and negative, far from the site of assembly.
This method of mapping, based on the diagrams of the architect Kiel Moe, illuminate that even small, prototypical structures such as Aguahoja I can have far-flung global footprints.